Theory of light-matter interaction in quantum materials
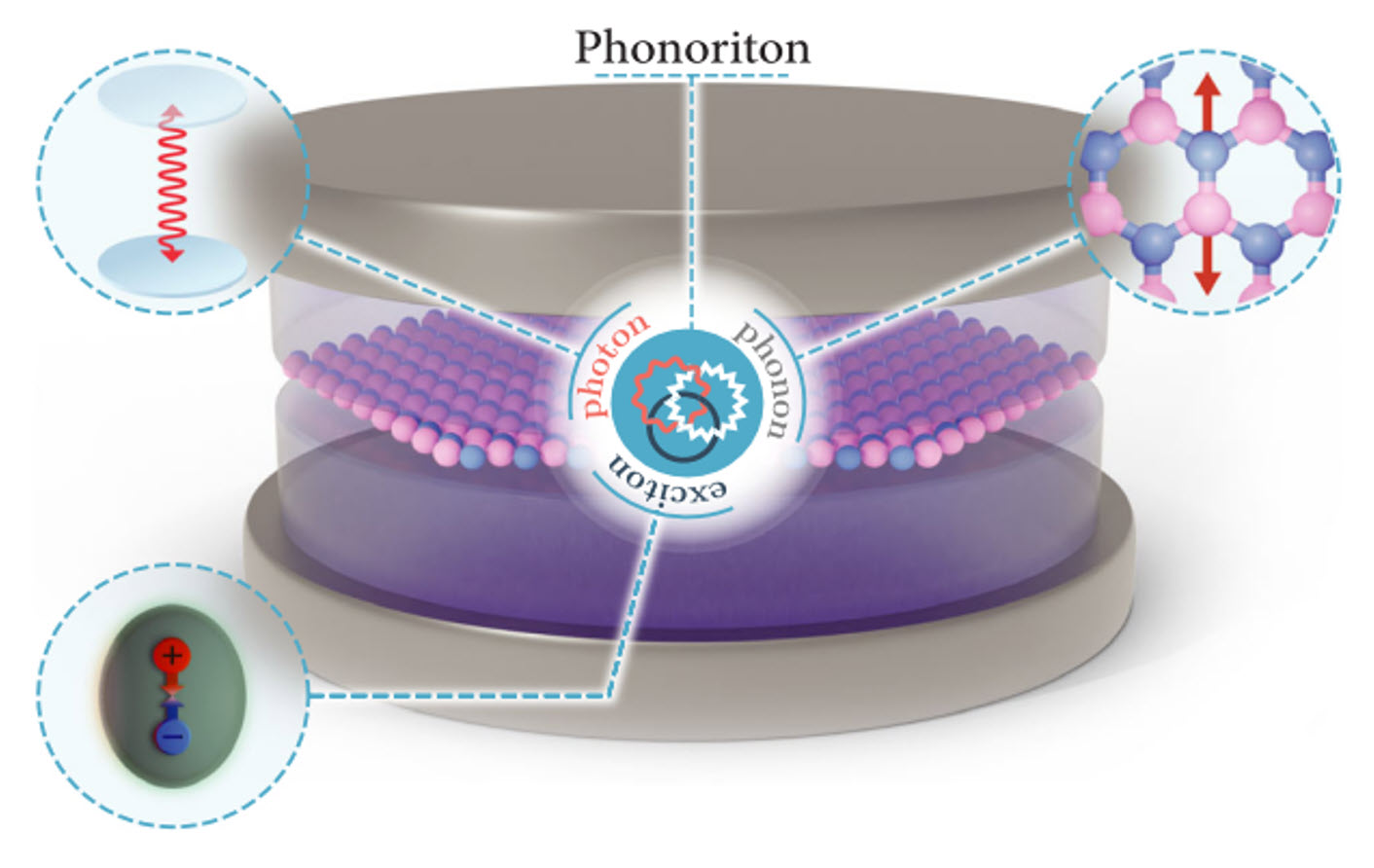
The strong interaction of a material with light can be exploited to alter the material properties altogether, in and out-of-equilibrium. By means of theoretical ab-initio techniques, in our group we investigate the diverse phenomena originating from strong light-matter interaction, such as light-dressing of electronic states [1], light-induced phase transitions [2] and light-driven charge migration [3]. Ab-initio methods, such as density functional theories and many-body perturbation theories, provide seamless connections across phenomena and material platforms while delivering reliable predictions.
Cavity materials engineering
A promising route to achieve strong light-matter interaction is to embed materials in optical cavities, as opposed to employing sophisticated laser sources to drive the materials out-of-equilibrium. The confinement of light in a narrow region of space, provided by the cavity, boosts the interaction between the photons and the microscopic constituents of the material. The boosted interaction can alter the collective behaviour of the material’s constituents unlocking, for example, magnetic, superconductive and ferroelectric phases [2] of a light-matter hybrid nature. The origin of the modified material properties can be understood in terms of a dressing picture, where the electronic (or nuclear) degrees of freedom of the material are dressed by the electromagnetic field and its fluctuations resulting in a light-matter state of hybrid character called polaritons [4, 5]. Along these lines, light can be identified as a tool to tailor materials properties to specific technological applications, ranging from sensing, information technology, or light-harvesting, among others.
In order to investigate these unexplored phenomena, in our group we develop ab-initio methodologies grounded in the low energy description of quantum electrodynamics (QED). Specifically we develop first-principle based model Hamiltonian, many-body perturbation theory methods and density functional theories extended to QED.
Time-resolved spectroscopies
Following light-driven ultrafast excitation dynamics in a material can yield invaluable insights on the out-of-equilibrium material’s behaviour which can transferred to the design of controllable light-responsive devices. The technological development of pump-probe spectroscopies has allowed scientist to achieve attosecond time-resolution in charge dynamics of both molecular and extended systems but the interpretation of the experimental data strongly relies on theoretical modelling. The theoretical description of these highly out-of-equilibrium systems poses both conceptual and technical challenges, in particular when an atomistic interpretation is needed. To address these challenge we use and develop theoretical techniques based on a combination of Time-Dependent Density-Functional theory and Non-Equilibrium Green’s function theory, heavily relying on high performance scientific computers. We are particularly interested in light-induced phase transitions [6], light-dressing of electronic states [7] and stabilising charge-migration mechanisms in bio-relevant molecular systems [3].
Two-dimensional (2D) materials
Graphene, transition metal dichalcogenides (and many more) are all examples of 2D materials, a class of materials which is arguably one of the most studied in the last decade, both because of their unusual electric, magnetic and optical properties and because they can be easily stacked into integrated multi-functional Van der Waals heterostructrures. What 2D materials have in common is the reduced dimensionality which makes the effective Coulomb interaction between charged microscopic constituents stronger than in the bulk counterpart. A prototypical signature of the stronger Coulomb interaction is the emergence of strong excitonic features in optical spectroscopies. Furthermore, many two-dimensional materials display properties that are linked to a non-trivial topology and exotic magnetic states. In our group we theoretically explore the possibility of using light, either from classical laser sources or exploiting optical cavities to control 2D materials properties on demand [4, 5]. In particular we are interested in the emergence of peculiar dielectric properties, such as dielectric hyperbolicity [1], non-trivial light-induced topology and exotic excitonic phases in so-called Moiré structures.
Link webpage - Simone Latini's Group
Contact
Simone Latini Tenure Track Assistant Professor simola@dtu.dk